In 1956, Britain built the world’s first commercial nuclear power plant, kicking off what some thought would be another industrial revolution. Clean and abundant electricity could have followed, reducing carbon emissions before most people had even realised global warming was happening.
Today, nuclear power accounts for around 15% of electricity production in the UK. A respectable number in global context, but nowhere near what it could have been. The technology has flopped relative to governmental expectations, purported technological feasibility, and popular support. What happened? Simon Taylor’s The Fall and Rise of Nuclear Power in Britain: A History is one of the few available accounts.
The doldrums
In 1946, following the Second World War, Britain was frozen out of the United States’ nuclear programme – American secrets would not be shared, even for the purposes of electricity generation. Despite the two countries’ close collaboration during the war, the US was hamstringing its closest ally.
Britain, therefore, chose to develop its own reactor, which could both create plutonium for a bomb and generate electricity via fission. The United Kingdom Atomic Energy Authority was established to make this happen, separate from the civil service and with independent powers to speed up decision-making.
In a nuclear reactor, a radioactive isotope (generally uranium 235 or plutonium 239) is bombarded with neutrons, which causes it to split into smaller atoms and release enormous amounts of heat and radiation. The nuclear fuel is immersed in a moderating fluid (a ‘moderator’), which slows down the neutrons produced by fission – slow neutrons are more likely to cause a sustained chain reaction than fast ones. While it does not touch it directly, the nuclear fuel is surrounded by a cooling fluid which transfers heat from the fuel to water. This cooling fluid heats up the water enough to boil it into steam, which rotates a turbine and generates electricity.
Britain’s first major decision was selecting which type of reactor to build. At the time, there were essentially three options available, differing in the materials used for the reactor’s cooling fluid and moderator:
Water-cooled reactor: Water is used as the cooling fluid and enriched uranium is the fuel. (Some versions of this design involve pressurising the water.) The main disadvantage of this approach is that enriching uranium is extremely expensive.
Heavy water reactor: Heavy water is the cooling fluid and enriched uranium is not required. However, producing enough heavy water to supply nuclear reactors would itself have been a tremendous industrial project.
Graphite moderator gas-cooled reactor: Also referred to as a gas-cooled reactor. This design had the double benefit of being fueled with cheaper ‘natural’ (unenriched) uranium, and using abundant, easily accessed carbon dioxide gas as its coolant.
The government chose to go with the gas-cooled reactor design.
The result was Calder Hall, the world’s first commercial nuclear power plant, which generated 65 MW of power with two reactors. Built in three years, it was successful enough that in 1956 the government made ambitious plans to add 2,000 MW of nuclear capacity in the next two decades (thirty times Calder Hall’s capacity). Later that year, the Suez Crisis sparked fears about Britain’s oil supply, and nuclear targets were increased further to 5,000 - 6,000 MW; one government official even proposed a 15,000 MW target. Yet almost 70 years later, in 2023, the UK's nuclear capacity is still at 5,900 MW.
The station was launched in dramatic fashion: Queen Elizabeth II arrived, continuing a long-standing British tradition of a monarch opening major infrastructure projects. The significance of the moment can hardly be overstated – the Lord Privy Seal described it as no less than “epoch-making”. The plant offered a tantalising glimpse of a new industrial revolution. One observer speculated: “It may be that… every new power station being built will be an atomic power station.” That sounds hubristic, but in the mid-twentieth century the transition from steam engines to combustion engines had barely finished, so British society was well-used to the idea that significant technological change was possible in a short space of time.
After a speech to the thousands of people present, the Queen flicked a switch, supposedly activating the flow of electricity from the reactor to the grid for the first time. However, the demonstration was reportedly fake; the three-metre arrow measuring the flow was moved by a man turning a handle rather than actually showing power flowing.
Taylor never really explores the economics of Calder Hall, and how a country that was basically broke after WWII could afford to build it. Perhaps it was justified by the prospect of developing a British nuclear weapon. Despite the falling price of coal and oil in the 1960s, then, the UK built six new stations with Magnox designs, which are graphite-moderated and gas-cooled, much like Calder Hall. These British-designed, gas-cooled reactors would serve the dual purposes of producing electricity and plutonium-239. They helped the UK build a nuclear arsenal and performed reliably for four decades.
In the late sixties, the old Magnox designs were working well and the UK’s nuclear capacity had expanded. The old constraints – the cost of enriched uranium & industrial capacity to produce heavy water – that had forced them into building gas-cooled reactors were no longer present. For the first time, the UK was faced with a genuine choice of reactor type; heavy water and water-cooled reactors were back on the menu.
However, Magnox designs were developed into a new concept: advanced gas-cooled reactors (AGRs). The Atomic Energy Authority invested heavily in this design, as its potential export was seen as a huge commercial and political opportunity. It was thought that the AGRs could refuel without being shut down, which would hugely improve their output and economics. On the other hand, no prototype had been built, the very designs themselves made construction challenging, the materials hadn’t been tested, and the stations were too dissimilar to allow modular construction (which would have saved money).
Despite these concerns, the UK’s state-owned electricity provider, the Central Electricity Generating Board (CEGB), forged ahead with the British-designed AGRs in what Taylor describes as a ‘truly awful decision’.
Problems began to mount as the first full-scale AGR was built in 1966, and eventually costs skyrocketed from an initial £89m to £344m (of which £150m was inflation). Had this mistake been rectified after the first warning signs, 20 years of dysfunction could have been avoided. Instead, the error was compounded. Despite repeated delays and cost overruns, Britain remained committed to indigenous reactor designs, hellbent on creating something they could export. And crucially, it turned out that the AGRs could not be refuelled without being shut down.
In 1973, by which time the AGR design was obviously problematic, the government moved to use yet another novel British design (the steam-generating heavy water reactor) instead of copying an existing design from another country.
Assessing this era, Taylor claims that being cut off from US designs was fatal. There was wishful thinking and delusion about the British designs and prospects for export. Ultimately the plants were put into managed decline.
Is it fair to criticise this desire for exports? The UK was losing much of its manufacturing sector to foreign competition, and large complex projects that required strong technical expertise are a fairly obvious high-value replacement for those industries.
A key conclusion many economists take from East Asian development is that to develop an industry well, a country must cultivate local knowledge and build things indigenously instead of importing all knowledge, expertise and labour. For example, Korean car firm Hyundai secured designs and technological know-how from Japanese competitor Mitsubishi, but went through the hard graft of actually building cars for itself and eventually caught up to the global technological frontier.
Perhaps the UK’s mistake was to bet on unproven technology instead of copying foreign designs already in operation. It’s tough to evaluate the feasibility of AGRs in hindsight, but Taylor’s account implies that they didn’t look promising, even at the time of development.
France, by contrast, after some early experiments with graphite-moderated, gas-cooled designs, licensed pressurized water reactor designs from US firm Westinghouse. This gave the French the best of both worlds: a design that actually worked (pressurized water reactors were already operational in the US) and the chance to develop expertise by building the PWRs themselves. From 1974 onwards, France ordered 40 reactors. And by 1985, two-thirds of French electricity generation was nuclear.
Électricité de France (EDF), the French equivalent of CEGB, was at the heart of this process. The UK never really achieved returns to scale by committing to a single reactor design (there were variations even within the AGR category). France, by contrast, standardised their reactor designs into three main types, so they didn’t have to waste time designing another plant every time they wanted to build one. (Three designs still seem like a lot, but it’s fewer than many other countries have managed.)
Relatedly, it has to be the same party making the same item – EDF’s unitary nature may well have helped with this, whereas the UK typically used many different companies, so knowledge was spread across many parties and not consolidated in a single firm.
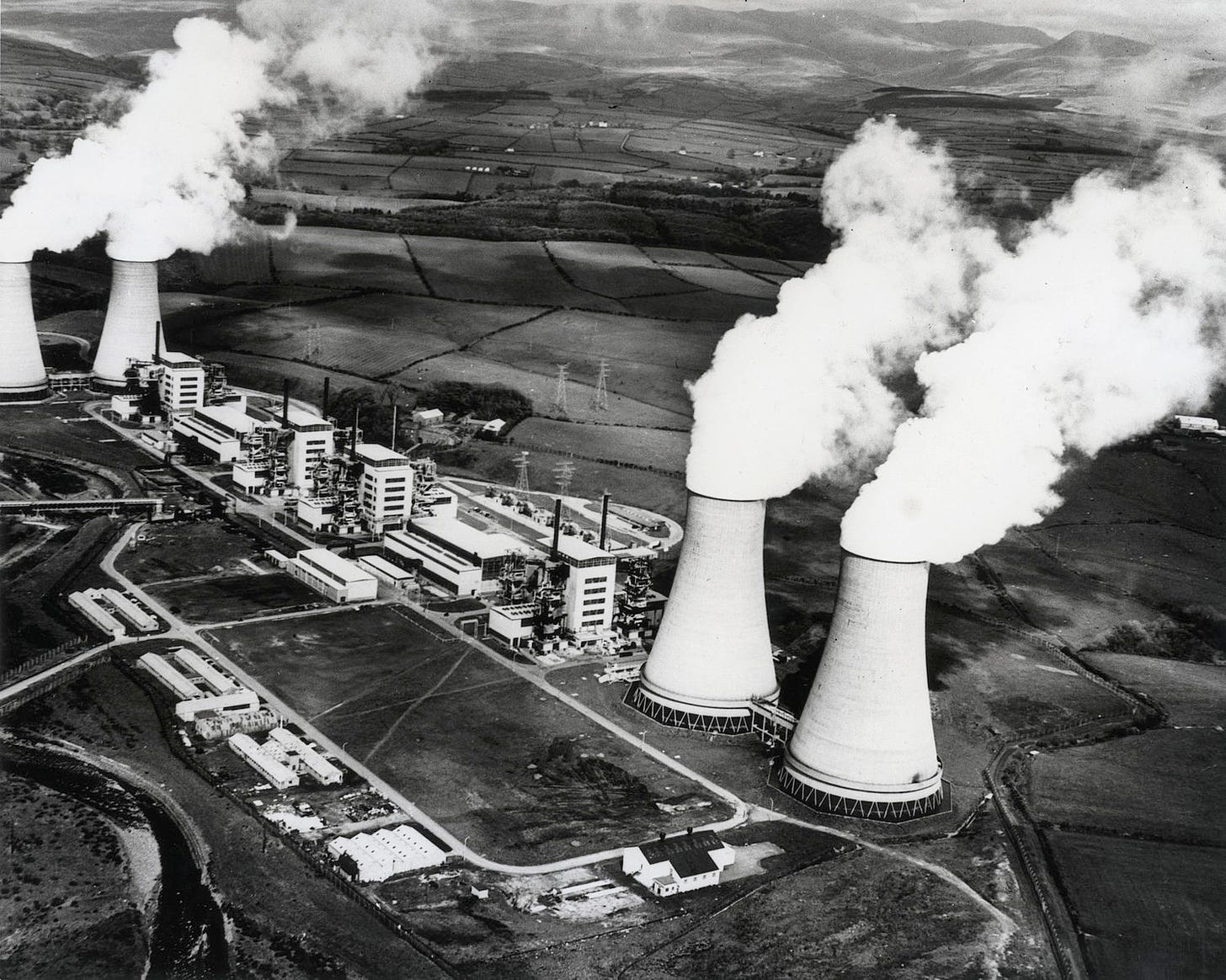
Nuclear strikes back (eventually)
Any investor who takes ownership of a nuclear plant is also committing to decommissioning it at the end of its life, and bearing that cost. For governments, developing nuclear weapons is an additional reason to build nuclear power plants – but for private entities, the decision is purely financial. In the late 1980s, Margaret Thatcher’s government decided to privatise the CEGB and transfer all electricity generation to private ownership, including nuclear plants. This opened the door to private companies operating & building nuclear power plants in the UK for the first time.
By this stage, the previous thirty years of nuclear boosterism were seeming increasingly misguided. One study1 pinned the decline of nuclear on the CEGB, which had underestimated the cost of nuclear for many years by ignoring R&D costs, assumed the costs of running a plant would be the same as for coal, overestimated the cost of competitor fuels, and underestimated decommissioning costs.
In the face of these financial uncertainties, privatisation was a mess. By 1989, the costs of decommissioning a plant were very high, estimated at anywhere between £8bn and £11bn. Coupled with the large investment required to purchase plants, the financial picture proved unattractive to investors and few wanted to take on these assets. The government backtracked on their plans quickly by announcing that they would keep the dozen or so Magnox reactors under government control. Months later, the privatisation of all other existing reactors was cancelled, and plans for new pressurised water reactors were shelved. This announcement came the day after Thatcher extolled nuclear power to the UN as a solution to climate change.
After decades of commitment to nuclear, the British government had now cancelled all new reactors. The economics had worsened: Decommissioning costs doubled for the Magnox stations, the cost of processing the spent fuel doubled too, and the remaining cash flows from the reactors appeared to be less than the cost of decommissioning. Arguably, Magnox reactors now had a negative financial value. In addition, coal-generated power appeared about half as expensive as that from the planned pressure water reactors.
The outlook for nuclear power in Britain was not good. The stations were put into managed decline under the control of two new government entities: Nuclear Electric for England and Wales, and Scottish Nuclear for north of the border. Then something strange happened: the companies performed really well. Taylor:
By focusing only on nuclear, with nowhere to hide losses or weaknesses, and with proper financial accounting, the managements of both [Nuclear Electric] and [Scottish Nuclear] achieved a remarkable turnaround in British nuclear performance. Both companies brought about dramatic improvements in productivity and costs, and hugely improved the performance of the unpredictable AGRs.
One of the most important measures of any power station is the load factor. Analogous to uptime in software, the load factor refers to the time the station is actually producing power. This becomes important for the economics of plants: Overall, fuel costs make up only 15% of the final price of nuclear energy.2 The remaining 85% or so pays back the cost of building & operating the reactor. Even if fuel costs change, the most important factor in making nuclear energy economical is the load factor. If a reactor is not generating electricity, it's not paying back the cost of building it – if that goes on for long enough, the reactor becomes unprofitable.
Ideally, a plant would run 100% of the time – but maintenance, inspections, and refuelling make that impossible. For two decades the UK had struggled to make its reactors perform reliably; load factor in the English and Welsh reactors was around 47%. Nuclear Electric, however, managed to achieve nearly 70% by copying best practices from around the world, especially that of US utility Florida Power and Light, benefitting from a spirit of openness in the aftermath of Chernobyl. They also cut their workforce from 13,000 to 9,500. The other entity, Scottish Nuclear, followed a similar model yielding similar results.
In March 1994 [Nuclear Electric] announced that the more modern nuclear stations, the AGRs, had achieved an average load factor of 73.9% compared with 73.2% for the fleet of [pressure water reactors] in the USA. For the first time the UK could claim a world-leading performance in nuclear power.
Dramatically improved financial outcomes followed these technical achievements, and the firms were merged into a new company, British Energy, which the government then privatised in 1996 at a share price of £2.03. The good times continued; by May 1999 shareholders would have received £1.14 per share in dividends, and the share price had increased to £5. The firm even began to expand into the US market.
Then, there were some warning signs of the firm losing its focus. Instead of power stations or fuel rods, the 1998-99 annual report apparently featured ‘the art of energy’ on its front cover. Shareholders were told that these images ‘conveyed a sense of innovation, creativity and vitality as well as expressing energy in the environment and how it touches our everyday lives.’ Indeed.
From 1999 onwards, the AGRs’ performance began to decline, and they broke down regularly (a development that is most unexplained by Fall and Rise). British Energy continued to pay its shareholders large dividends but took on debt to fund further acquisitions. This ultimately left them short of cash for any electricity price fluctuations. By 2002, lower electricity prices forced the company into insolvency and the government, now its senior creditor, took it over in 2002. (Eventually, it was sold to EDF in 2009.)
Perhaps the most confusing element of this is the performance of the AGRs – the British design that Taylor argues they never should have pursued and which was excoriated at various points in the book. This reactor design was supposedly awful, their eventual breakdowns undermined British Energy’s financial results, and yet at one point, the plants’ stellar performance drove huge profits.
Taylor argues that at the dawn of the 21st century, nuclear power in Britain was the victim of “hubris, financial mistakes and some bad luck”; nuclear hadn’t worked under public ownership, and now in private ownership too.
Third time lucky?
Despite its torrid record, a third act for UK nuclear began in the mid-2000s. In previous decades, the case for nuclear energy was grounded in energy security and economics, but political attention had shifted to climate change. Following a third general election victory, Tony Blair was confident enough to propose nuclear energy as a way to replace the UK’s fossil fuel plants and reduce emissions. In 2006-2008, the government released various whitepapers committing them to nuclear power. In addition, the Climate Change Act bound the UK to cut carbon emissions by 80% by 2050, which also boosted the case for low-carbon energy sources like nuclear.
Work then went into choosing the location of the new plants: in early 2009, eleven possible sites were nominated. However, the nomination of these sites, even after an extensive selection process, did not guarantee that nuclear plants would be allowed to be built there. Planning permission still had to be granted by the government.
A fundamental assumption of the government was that there was lots of business interest in new nuclear plants in Britain – often from large state-owned energy firms like EDF and Vattenfall. Though early signs indicated that was the case, ultimately luck conspired against the government: the Fukushima disaster in 2011 led to two German firms withdrawing from consideration, and the post-2008 financial environment was grim. Despite this, EDF, having bought British Energy, wanted to build more nuclear plants, including a third plant at the existing nuclear station Hinkley Point. Much of the latter part of Fall and Rise is focused on this third plant, known as Hinkley Point C.
EDF’s choice of the latest European design – the European Pressurized-Water Reactor (EPR) – for Hinkley Point C made sense initially. The design was going to be implemented in Finland, France, and China first, and any major difficulties should have been ironed out before the UK build. However, those first plants ultimately suffered multi-year delays and cost overruns, undermining the economic case for EPRs.
EDF was also negotiating with the government on a contract for difference (CfD) in 2013. This was essentially a price guarantee on the electricity produced by the future plant. This price was supposed to be determined by an auction, but EDF was the only bidder. The eventual price of around £92/MWh was far above the average power price that year of £50/MWh, but the government was predicting that other low-carbon electricity would be as or more expensive by the time the plant was active. At the time, prices for onshore and offshore wind were at or above this level, so this was reasonable. On the other hand, the price of renewables was falling – a trend that continued sharply in the 2010s, for solar power in particular.
Simply using the word ‘electricity’ can obscure the distinction that different energy sources cannot provide electricity in any and all circumstances. Nuclear power is difficult to turn on and off, so is well suited to providing a stable baseload, while renewables are highly intermittent – and natural gas can relatively easily be burned to generate electricity at short notice. The ‘price’ of electricity is not a trivial or wholly well-defined concept. For this reason, it might sometimes make sense to ‘overpay’ for electricity; equal capacities of different power sources are not interchangeable.
This government overpaid through the CfD because nuclear power has become incredibly expensive to build. The construction cost for Hinkley Point C, when the deal was announced, was £16bn, with a maximum cap of £24bn. That would make it one of the most expensive power plants ever produced – China’s Three Gorges project was more expensive, but its capacity was 22GW, around 7x that of Hinkley. Megaprojects like Hinkley, Crossrail, and HS2 have a habit of overrunning, due to a range of factors, from non-standard design and technology to changes in scope and conflicting interests among participants. Furthermore, the UK didn’t exactly have an existing supply of nuclear construction experts (a problem that France has faced more recently when trying to upgrade its own nuclear fleet).
One way to solve the bad incentives of megaprojects is to shift the risk to a party willing to accept it. The CfD provided one aspect of protection; EDF knows what price it will get for electricity once the plant comes online. In addition, the government underwrote 65% of the overall project costs via guaranteed loans (if the company defaulted on its loans, the government would pay off the creditors). This seems reasonable considering how important the project is for the country. But Taylor is critical of how the deal was structured:
It is a very inefficient way of covering the main risk of the project, which is the construction risk. Instead of explicitly covering or at least mitigating that risk, it provides a 35-year price promise that potentially overcompensates the investors for a risk that will have dropped out by the time the station operates. How else could the deal have been struck?
Note that at the point the book was published in 2013, the final investment decision about Hinkley Point C still hadn’t been taken. In fact, it would be 2016, almost a decade after the government began its new nuclear push, before EDF made a firm commitment to build this new plant.
Perpetual take-off
Since the publication of Fall and Rise, delay has been the watchword of nuclear power in Britain – and much of the rest of the world. At the time of writing, Hinkley Point C’s current completion date is 2026, twenty years after this generation of nuclear plants was proposed – but there are rumours of delays until 2036. EPRs are looking like a bad bet; the Finnish plant only recently entered service, a decade late, and the French one is similarly delayed by 10 years. The Chinese did get there first in 2018, but again with delays and cost overruns.
The record of the EPRs is so bad, in fact, that it is used to question the whole idea of costs falling as you do many iterations. Despite the poor record of this reactor type, Taylor points out that it would be even worse to cancel Hinkley Point C now, given the millions – billions, in fact – that have already been spent on the project.
There are a few other British plants in the works, all part of the original ten sites approved in 2008, but they are not nearly as far along. Bradwell B is still at the feasibility stage. Sizewell C’s financing is still not in place, and construction is estimated to take a further decade.
What should we conclude from nuclear power’s story in Britain?
First, constructing large one-off projects is phenomenally difficult and expensive in developed countries today. There are many reasons for this, like the use of non-standard parts and ‘scope creep’. One of the themes of the Transit Costs Project is that many Anglosphere countries have extensively outsourced their expertise in construction to consultants, so perhaps that is at play here too. I don’t want to be unambitious, but it’s difficult to see these costs falling without radical changes to how we undertake large projects. In the particular case of nuclear, Brian Potter has suggested that extensive regulations are part of what makes plants expensive to build, so perhaps that is an area for reform.
Second, government help seems like a necessity for any of these large plants to succeed. Cost overruns and delays make it hard to imagine private actors wanting to deploy capital in this industry, at least if they have sole responsibility for the financial success of the project. I am not instinctively a fan of government subsidies and loan guarantees, but it’s hard to imagine a nuclear future of any kind without them.
In the future, small to medium-sized reactors, should they ever materialise, offer a real opportunity for standardisation (reducing cost) and distribution around the country (reducing the strain on the grid). However, as yet they are unproven. Fusion offers another potential solution, and one can only hope that Helion and other firms can finally commercialise it.
A previous essay on the Fitzwilliam argued for the removal of the ban on nuclear power in Ireland – in part because Ireland could serve as a nuclear ‘regulatory sandbox’ for the rest of the world. Ireland could avoid the mistakes other countries have made in regulating nuclear, and strike a better balance between red tape and innovation. But the British case demonstrates that, even absent outright government obstruction, nuclear power is a tortuously difficult technology to implement.
Fergus McCullough is managing editor of the Fitzwilliam. You can follow him on Twitter here.
A Study of the Privatisation of the Electricity Supply Industry in England and Wales, Alex Henney, 1994.
By contrast, the cost of fuel accounts for between 50% and 90% of the costs of coal- and gas-fired power plants.
This is a great piece. The UK almost got it right when it constructed Sizewell B, a PWR of Westinghouse 4 loop design, coming online in the mid 90s. Had it continued with the same design the state of UK nuclear would be much better.
It is always tricky to go back into the past and second-guess decisions that had to be made without the benefit of hindsight. One of the reasons why the AGR turned out to be a dead end is the fact that it can't undergo life extension unlike the standard pwr and bwr designs. But back when the UK committed to the AGR build-out, the possibility of life extension wasn't well understood yet.
Having already committed so much to Hinkley point C, Sizewell C surely makes sense. However I hope that the UK will make room for nuclear that is not from EDF. For large reactors, ABWR from Japan would be an excellent choice. For small reactors, maybe it's time to give local champion Rolls Royce a boost.
Great detail, thanks for the piece